Over one third of the world’s population resides within 100 km of a coast, and this is expected to increase to 50 % by 2030 (Bilkovic et al., 2017). This places a significant proportion of the globe in the direct path of coastal hazards including erosion, flooding, and extreme events such as hurricanes and tsunamis. For decades, hard coastal protection structures (e.g., seawalls, breakwaters) have been implemented to protect against such hazards. However, these structures have also caused adverse environmental impacts, including biodiversity loss from habitat replacement, and coastal wetland loss because of changes to critical sediment supplies (Bilkovic et al., 2017).
Coincidentally, the very ecosystems that have been historically degraded by shoreline armouring can themselves provide coastal protection. Vegetated coastal ecosystems, such as salt marshes, act as buffers to coastal hazards, with demonstrated reductions in incident wave heights of up to 72 % (Rahman et al., 2019). These environments are also self-repairing and can adapt to rising sea levels through sediment deposition (ecosystem engineering), meaning they can provide protection in a changing climate.
The effective functioning of coastal vegetation for wave attenuation and erosion protection has incited an interest in its use for coastal protection infrastructure. With nearly 10 % of the total saltmarsh extent in Canada located along the St. Lawrence’s estuary and gulf—309 km2 of marsh (Rabinowitz et al., 2022)—these environments could play a critical role in long-term coastal protection strategies for the region. Efforts are currently being made to understand just how much protection these ecosystems can provide, how we can begin to implement them on the ground, and in what settings they may be most effective.
This article aims to provide an overview of nature-based solutions (NbS) for coastal protection, introduce the key controllers of wave attenuation by vegetation, and explore lessons learned from experimental studies of NbS performed by researchers from the University of Ottawa (Ottawa, Canada) and the Insitut national de la recherche scientifique (INRS) (Québec, Canada), in collaboration with the National Research Council (NRC) (Ottawa, Canada).
Nature-based Solutions and Coastal Marshes
Overview of Coastal Marshes
Coastal marshes are intertidal grasslands, often found along protected shorelines or at the edge of estuaries (Barbier et al., 2011). They are characterized by low species diversity and distinct zonation of plants between high and low marsh regions (Barbier et al., 2011). In the context of coastal protection, saltmarsh plants can stabilize the soil with their roots and capture sediment during tidal flooding, increasing the marsh platform elevation to reduce erosion even under rising sea levels. Vegetation also acts as flow resistance, exerting a friction force on the water, which reduces the velocity and height of incoming waves (Figure 1). Frequent tidal inundation and nutrient uptake through enhanced sediment deposition also make these environments effective carbon sinks and highly valuable for climate change mitigation (Barbier et al., 2011).
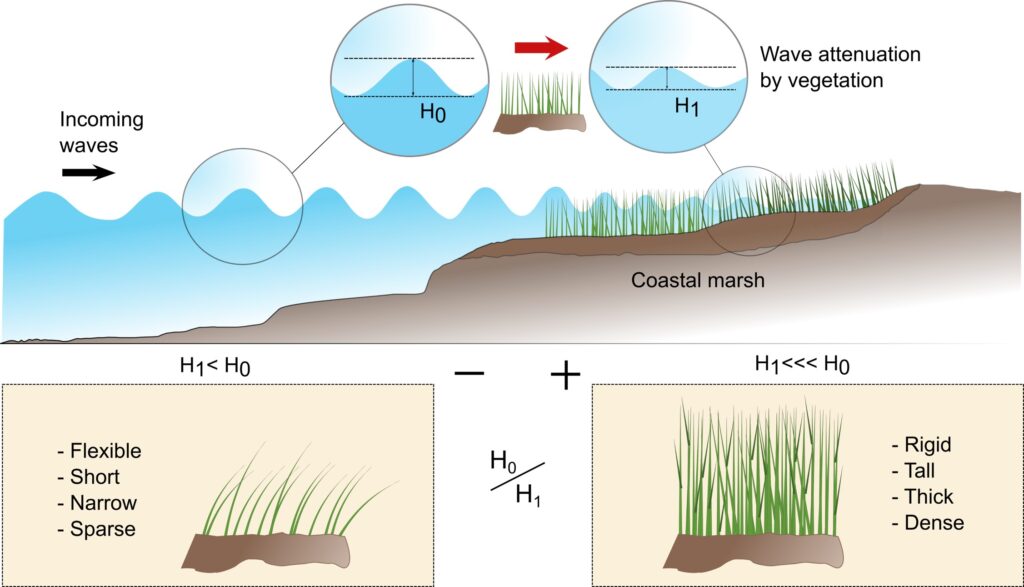
Flow resistance (friction) induced by vegetation reduces the height and speed of waves moving onshore (H1 < H0). The degree of wave height reduction (expressed here as the ratio of incoming wave height, H0, over the reduced wave height, H1) is impacted by vegetation parameters including flexibility, plant height, stem density, and plant thickness.
Coastal Protection with Vegetation
Coastal marshes exist in many forms in nature, ranging from vast complexes, enclosing tidal creeks, to narrow bay-fringing marshes. Similarly, they may be used in many forms for coastal protection. Large-scale managed coastal realignment projects support coastal protection through restoration of vast expanses of wetland area. On a smaller scale, marsh vegetation may be directly incorporated into engineered coastal structures often referred to as “living shorelines” (Figure 2).
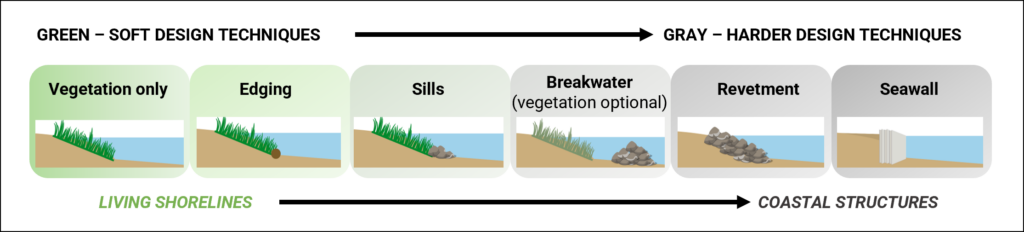
Living shorelines consist of vegetation planted on a constructed mild slope, with the primary purpose of providing additional wave energy dissipation. The addition of edging, sills, or an offshore breakwater shift toward gray infrastructure but remain within the umbrella of living shoreline design.
Some of the key factors determining the level of coastal protection provided by NbS with vegetation (e.g., living shorelines) are vegetation submergence (water depth), marsh canopy height, plant biophysical properties (morphology, stem diameter, flexibility), and flow energy setting (waves, currents) (e.g., van Veelen et al., 2020). Flexibility plays an important role, as the bending or passive motion of vegetation with the water can reduce flow resistance and thus overall wave energy dissipation. Many of these factors are highly region-specific, depending on the characteristics of native vegetation species as well as local environmental conditions. This emphasizes the need for region-specific studies and guidelines for NbS design.
Laboratory Experiments for Coastal Marsh NbS
Experimental studies, or physical models, of NbS aim to recreate natural coastal environments in a laboratory setting to investigate wave-vegetation interactions in a more controllable and accessible location than the field. These studies can support the development of design guidance for NbS, allowing quantification of coastal protection services considering different plant species, coastal settings (tides, wave conditions), and vegetation configurations. For the present study, a multiscale experimental approach was used to investigate the coastal protection services associated with Sporobolus species in a living shoreline design (Figure 2, leftmost panel, and table 1).
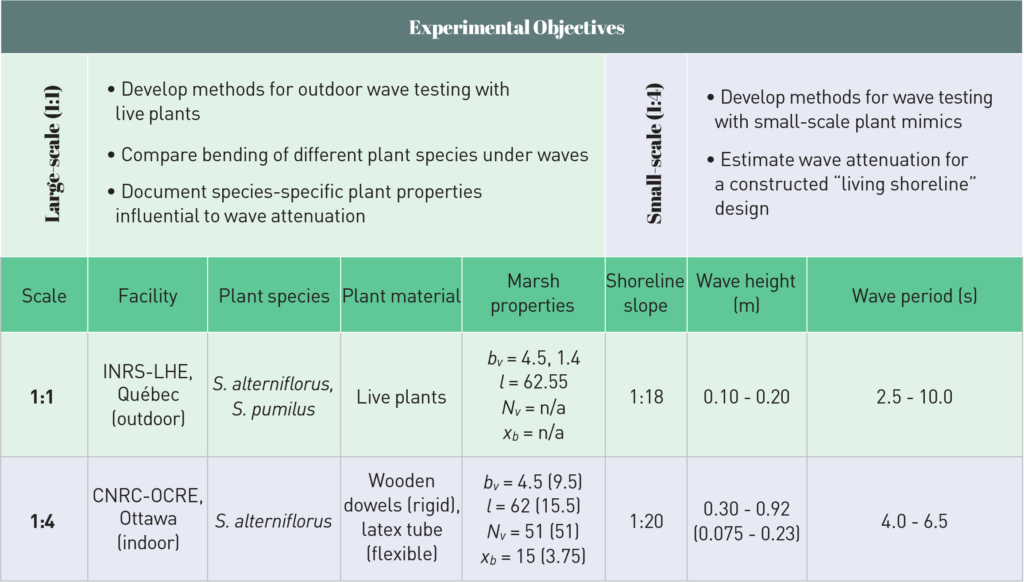
Acronyms for marsh properties: bv = plant stem diameter (mm), l = plant stem length (cm), Nv = number of stems per unit area (stems/m2), xv = marsh length (m) in the direction of waves. Live vegetation properties are averages. For small-scale experiments, prototype-scale values are reported with actual model values in brackets.
Large-scale wave tests with live Sporobolus alterniflorus (smooth cordgrass, previously Spartina alterniflora) and Sporobolus pumilus (Spartina patens or saltmarsh hay) plants were conducted in summer 2021 at the Laboratoire Hydraulique Environnemental (LHE) of the INRS in Québec. Sporobolus species were selected for testing as they are both native to Canada and widespread globally. Within Canada, the native distribution of S. alterniflorus is primarily limited to the east coast, whereas S. pumilus are also found along the west coast (Natural Resources Conservation Service, 2021). Live plants were sourced from a natural marsh located near Trois-Pistoles, province of Quebec (Figure 3). In nature, flood-tolerant S. alterniflorus dominates low marsh areas, while S. pumilus thrives in the high marsh. The two species were planted in the experimental facility accordingly.
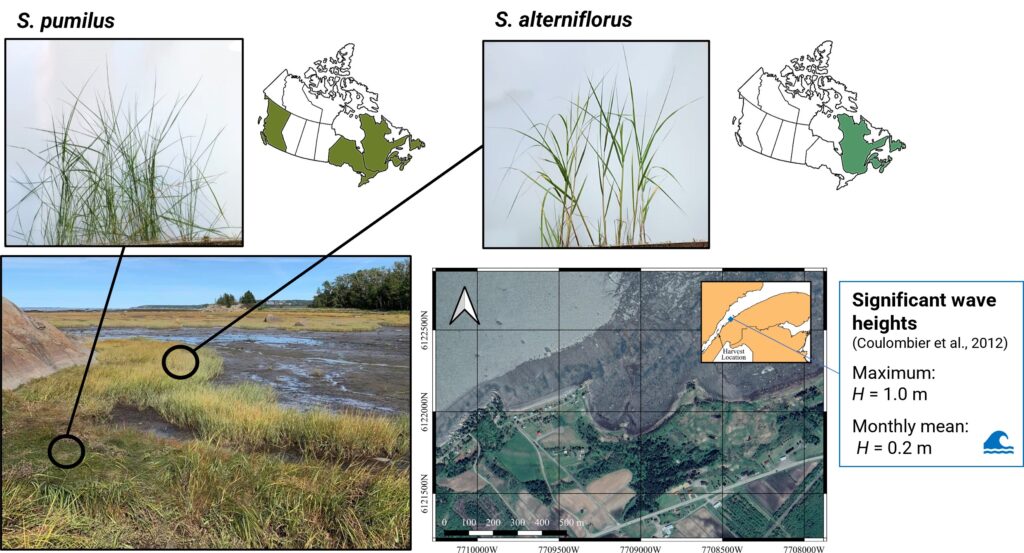
Live vegetation used in large-scale wave tests (S. pumilus, S. alterniflorus) including their native distribution in Canada, plant harvest location near Trois-Pistoles, and approximate wave climate for the site (Coulombier et al., 2012). Distribution maps adapted from Natural Resources Conservation Service (2021).
Experiments with live vegetation were performed to obtain fundamental knowledge of plant biophysical properties, while investigating differences between the two species and observing how these differences might impact coastal protection functions. The latter aspect was investigated using underwater video cameras (Figure 4), observing movement of the plants as they were subjected to different wave and water level conditions.
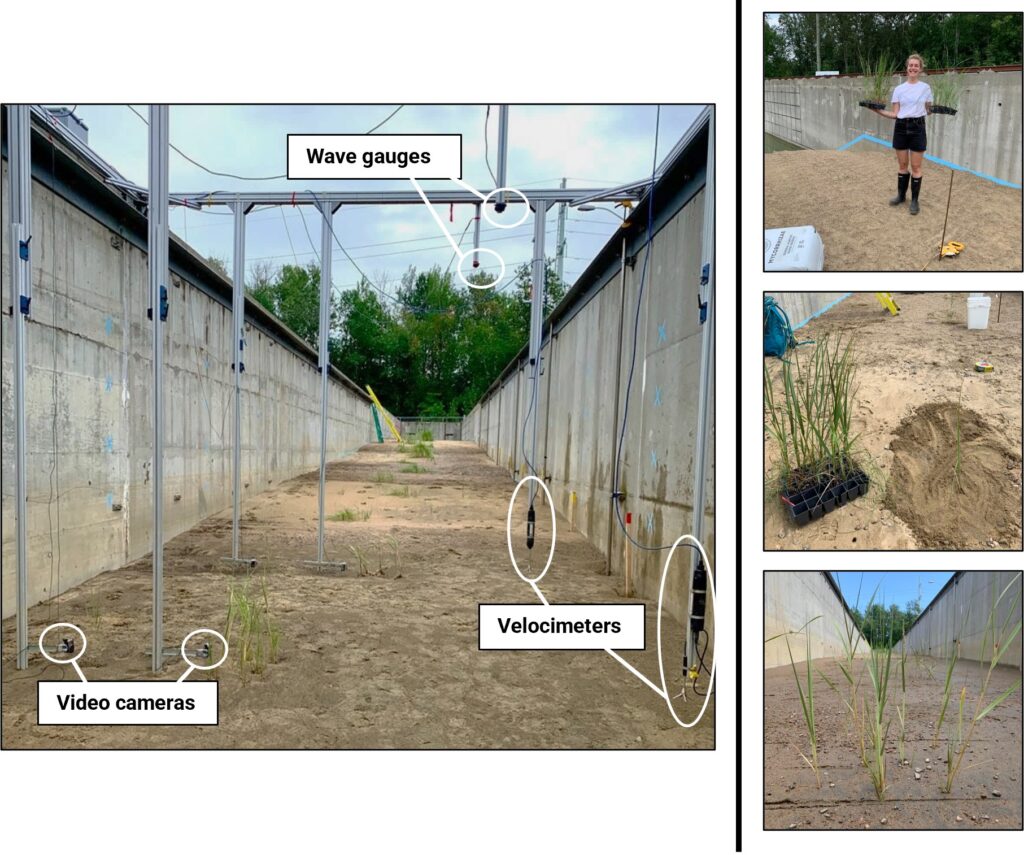
Live plants were transplanted directly into the wave testing facility and, for three weeks, were watered and monitored to allow them to establish in the soil before the experiments.
The small-scale laboratory experiments conducted for this project aimed to investigate the wave attenuation provided by a living shoreline structure vegetated with S. alterniflorus (Figure 5). The structure built in the NRC-OCRE laboratory represented a 1:4 scale model and used simplified plant mimics to represent S. alterniflorus. Mimics were developed using the measured properties from live vegetation experiments and included both a flexible (silicone rubber tubing) and a rigid material (wooden dowel).
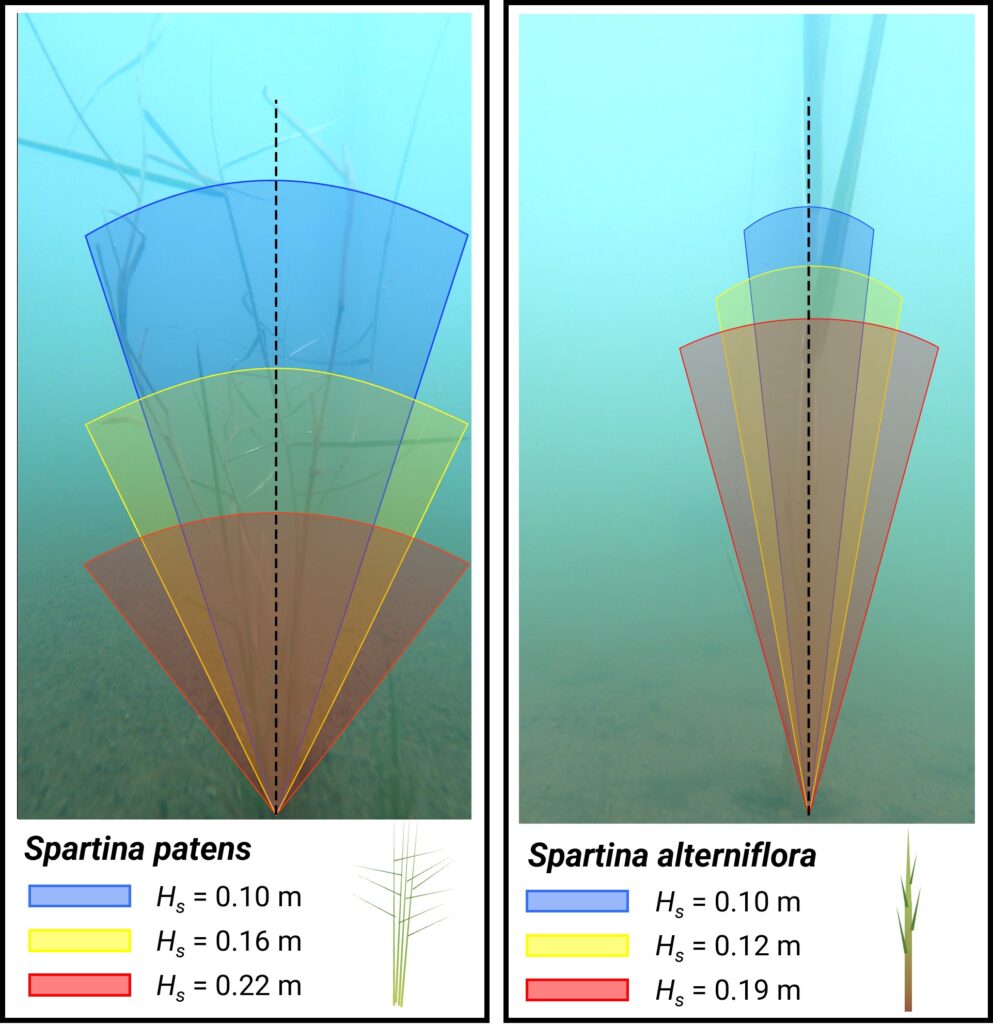
and S. alterniflorus stems, corresponding to sample wave conditions from large-scale experiments; obtained from underwater video footage. Results
are from irregular wave tests with a wave period (Tp)
of approximately 2.5 s.
The plant diameter and meadow density were selected to preserve flow-stem interactions and obtain a vegetation volume fraction equivalent to that of a prototype-scale living shoreline with staggered plantings spaced at 15 cm. This produces a meadow with a lower plant density than would be expected in a natural marsh in Atlantic Canada (100–350 stems/m2, Virgin et al., 2020), instead representing a newly constructed or planted marsh scenario. The meadows of flexible and rigid plant mimics underwent wave testing separately, to investigate the influence of flexibility in small-scale experiments with NbS. An overall vegetation field length of 15 m (3.75 m in the laboratory) was tested under a range of increasingly energetic waves, with wave heights between 0.3 m and 0.9 m (0.075–0.23 m in the laboratory), and with two water depths (partially submerged and fully submerged vegetation conditions).
Outcomes
Very few experimental studies of NbS use live vegetation, due to challenges with plant sourcing, transportation, and care in laboratory facilities. Alternatively, the majority have used plant mimics of various materials and complexities to represent live vegetation in the lab. The use of mimics not only eliminates the complexities associated with live plant care, but also allows testing at model (reduced) scales and offers better reconfigurability (i.e., many design scenarios can be tested efficiently). However, some accuracy may be lost in these studies, as vegetation is reduced to a simple morphology with uniform material properties. Thus, the use of live vegetation in laboratory studies is essential to investigate the fundamental behaviours of marsh vegetation in coastal settings (i.e., with waves). Live plant studies realistically capture vegetation structure and allow for comparative testing of different plant species, capturing inter- and intra-species heterogeneity in plant properties, as well as phenomena such as stem bending and motion.
Overall, the use of a multiscale approach in this project benefited from both experimental settings, supporting a more holistic approach to understanding NbS and coastal protection by Spartina species. Some key findings from the experiments are presented below.
Plant Properties and Wave Attenuation
The plant properties measured during live vegetation experiments highlighted key differences between S. alterniflorus and S. pumilus that may influence their respective role in NbS design. S. alterniflorus plants were characterized by an individual semirigid stem with several wide, flexible leaves, whereas S. pumilus plants consisted of a collection of several flexible, narrow stems, with long, flat leaves. These differences, particularly in stem flexibility, led to significant differences in plant motion when exposed to waves: S. pumilus plants consistently exhibited more stem bending than S. alterni florus under comparable incident wave conditions (Figure 5).
Coastal vegetation often adapts one of two strategies when faced with flow forcing: “avoidance” or “resistance.” Plants that “avoid” flow are often small, highly flexible, and may bend or move passively with waves to minimize stress. Plants that “resist” may be larger or more rigid to withstand flow forces. Both strategies result in a trade-off for coastal protection. Avoidance plants provide little resistance to flow and thus less wave energy dissipation. Resistance plants provide more potential for wave dissipation but are also more susceptible to damage in high-energy environments. In fact, there are benefits to combining both types of species in coastal protection strategies, as the resistance species provide greater protection below their threshold for breakage, and avoidance species may provide prolonged protection with a greater chance of survival (Schoutens et al., 2020).
With respect to the Sporobolus spp. observed in this project, S. pumilus appears to take more of an avoidance strategy, and S. alterniflorus a resistance strategy. This indicates that we can potentially derive benefits from the combined attenuative capacity of S. alterniflorus and the resilience of S. pumilus, when paired together in coastal protection strategies.
NbS & Living Shoreline Design
In the small-scale experiments, S. alterniflorus mimics were used to directly investigate the wave attenuation associated with a living shoreline structure. Sample results from these experiments are shown in Figure 6. Overall, tests with flexible and rigid plant mimics produced very similar results.
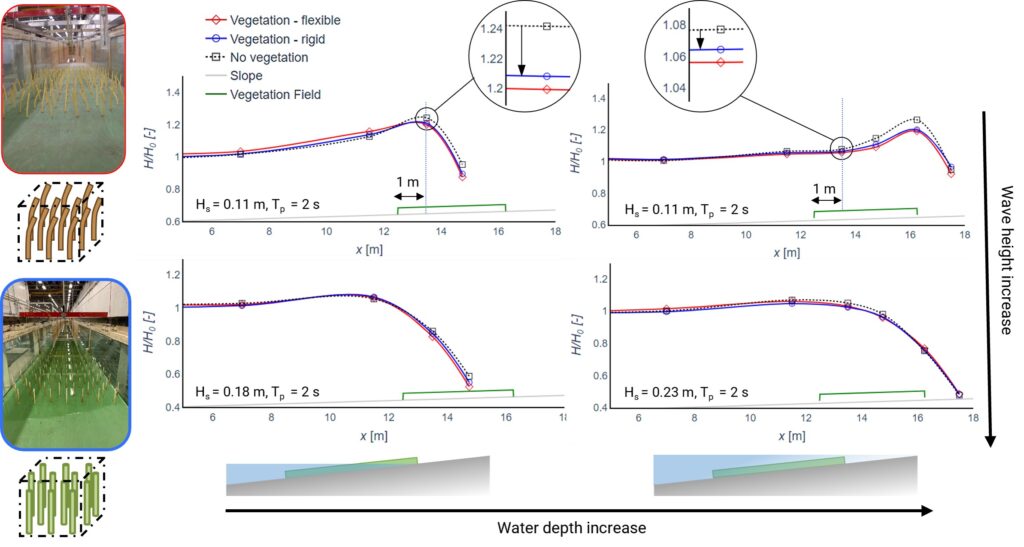
Sample results of wave height evolution over a scaled living shoreline model, using mimic vegetation (rigid and flexible) to represent S. alterniflorus.
Living shorelines often consist of a sloping structure vegetated with coastal marsh plantings. The slope alone can alter incoming waves through processes such as shoaling (wave height increases as water depth decreases), and wave breaking. The latter occurs if the wave becomes too steep, as the water becomes increasingly shallow. Therefore, when investigating the performance of vegetation in NbS designs such as living shorelines, it is important to consider the relative contribution of the vegetation to wave energy dissipation, versus the effect of the slope itself.
In the current experiments, the largest attenuation due to vegetation occurred for the smallest incident wave conditions (lab : 0.075 m, real-world: 0.30 m), with a maximum wave height reduction of 11–19 %, depending on the wave period, compared to the bare slope. With increasing wave heights, wave attenuation due to vegetation decreases in comparison to attenuation due to slope-induced wave breaking: for the largest waves tested (lab : 0.23 m, real-world: 0.92 m), the vegetation provided almost negligible energy dissipation compared to the slope alone. For design purposes, it is expected that wave height reduction could be improved through increasing the vegetation density; however, this may not yield improvements for cases of large incoming waves, due to the dominance of slope effects.
The results from these experiments also demonstrated the importance of vegetation placement relative to the still-water level (SWL). For cases where the SWL was located at the end of the vegetation field (Figure 6, left), the first 1 m (or ~25 %) of model vegetation has a measurable impact on the waves. However, when the SWL is raised higher (Figure 6, right) vegetation effects are not evident until several metres into the canopy. This indicates that the total marsh length on a living shoreline may not be equivalent to the length of vegetation that will produce wave attenuation effects, and this should be considered in their design.
Overall, the results from model-scale experiments must be interpreted keeping in mind their limitations arising from the use of mimic plants and downscaling effects.
Conclusions and Outlook
The incorporation of native marsh vegetation (such as Sporobolus pumilus and Sporobolus alterniflorus on Canada’s east coast) in coastal infrastructure has the potential to provide adaptive coastal protection in the context of climate change. To improve the rate at which these solutions are implemented on the ground, more studies like those presented here are needed, to support the development of design guidance for coastal marsh–based NbS. While this research is being conducted worldwide, region-specific studies are also integral. Based on the findings and limitations of this project, the following future studies to support NbS implementation in Canada are recommended:
- Identify thresholds for vegetation damage, and potential associated reductions in coastal protection.
- Investigate seasonal effects, including the presence of ice and vegetation biomass fluctuations.
- Consider other native coastal marsh species, particularly for the Pacific coast of Canada where S. alterniflorus is considered invasive and thus restricted from NbS design.
- Develop pilot-scale living shoreline projects, to test hypotheses derived from laboratory models.